Energy efficiency in high-speed train operation
Posted: 4 August 2010 | | No comments yet
Reduction of energy consumption has become a global concern, and the EU is committed to reducing its overall emissions to at least 20% below 1990 levels by 2020. In the transport sector, measures are focused on planning, infrastructure, modal change, the renewal of vehicles and also programmes for efficient driving.
Factors such as the low friction wheel-rail that allows coasting for long distances, electric traction which may allow the use of renewable energy, a capacity to transport large numbers of people and the possibility of adapting the number of coaches to demand, make the railways an efficient mode of transport. Additionally, railway transport is a guided path transport mode which allows the existence of electrification and facilitates traffic control and the automation of driving.
Reduction of energy consumption has become a global concern, and the EU is committed to reducing its overall emissions to at least 20% below 1990 levels by 2020. In the transport sector, measures are focused on planning, infrastructure, modal change, the renewal of vehicles and also programmes for efficient driving. Factors such as the low friction wheel-rail that allows coasting for long distances, electric traction which may allow the use of renewable energy, a capacity to transport large numbers of people and the possibility of adapting the number of coaches to demand, make the railways an efficient mode of transport. Additionally, railway transport is a guided path transport mode which allows the existence of electrification and facilitates traffic control and the automation of driving.
Reduction of energy consumption has become a global concern, and the EU is committed to reducing its overall emissions to at least 20% below 1990 levels by 2020. In the transport sector, measures are focused on planning, infrastructure, modal change, the renewal of vehicles and also programmes for efficient driving.
Factors such as the low friction wheel-rail that allows coasting for long distances, electric traction which may allow the use of renewable energy, a capacity to transport large numbers of people and the possibility of adapting the number of coaches to demand, make the railways an efficient mode of transport. Additionally, railway transport is a guided path transport mode which allows the existence of electrification and facilitates traffic control and the automation of driving.
However, a more efficient railway system is possible. Strategies to reduce energy consumption can be focused on infrastructure, vehicle design and traffic operation.
Infrastructure design during strategic planning should consider those factors that would contribute to a reduction in electrical losses, increase the use of regenerative braking and improve the efficiency of on-line traffic control with the installation of communication infrastructure. Some appropriate strategies include the installation of reversible traction substations for DC feed railways, designing the electrical network to improve the interconnection of electrical sections, new catenaries designs, the possibility of increasing the line voltage, or the use of energy accumulation devices. The efficient design of the track, avoiding where possible, speed restrictions under the maximum speed of the line, is also critical.
The design of the vehicle – in particular the train aerodynamics, the efficiency of the traction system and the reduction of the mass by modifying the train design or by using new light materials – has a direct impact on energy consumption. The contribution of the consumption of auxiliary systems to the total consumption of the train must also be considered. According to the results of onboard measurements in high-speed trains in Spain, this contribution is around 11% of commercial service consumption. These consumptions can be reduced by means of an optimised temperature control of the air conditioning, or the installation of a specific parked train mode to minimise energy consumption when there are no passengers on the train. An analysis of the impact on energy consumption of the installation of onboard energy accumulators, taking into account the characteristics of the accumulators and the increased mass to be transported by the train, could also produce significant results. As stated above, communication and control devices such as driving advice systems or efficient ATO systems, may improve the efficiency of the control of the train in real-time. Onboard energy metering is also necessary to determine the actual consumption and then to apply successful strategies to reduce it. Finally, the modularity of trains makes it possible to adapt the capacity of the train to passenger demand, and the increase in the number of seats in each coach in new train designs also contributes to a reduction in energy consumption per passenger.
Traffic operation
Both infrastructure and vehicle strategies are long-medium term decisions and involve important investments. On the other hand, different strategies, focused on rail traffic operation, can be applied in the short-term or even in real-time. Given the current infrastructure, and the vehicles now in service, rail traffic operation may now take into account energy efficiency goals in addition to operational and commercial ones.
Strategies focused on traffic operation can be divided into three types: planning, ecodriving design and real time traffic control.
During traffic planning, the adjustment of the service offered to passengers to the actual demand is fundamental to the achievement of desired levels of train occupancy. Of course, a compromise must be found due to the fact that a railway is frequently considered to be a basic service offered to citizens and that society feels there is a need for minimum rail services, even if these are not justified by demand. (This is particularly the case for commuter trains or metro lines). The efficient design of timetables may also reduce energy consumption. When timetables are designed, the minimum running time (flat out) is increased by a time margin, known as ‘recovery time’. This makes it possible to make up time in the event of delays. This recovery time is needed if the rail system is to comply with punctuality requirements and can also be used to reduce energy consumption if the train is not delayed. In addition, the way the time margin is distributed during the trip should take into account, not only punctuality criterion according to the probability distribution of delays along the line, but also energy efficiency criterion. Models have also been developed for the design of timetables that maximise the regenerate braking energy transfer between trains in the same electrical section, and have been implemented and measured in metro lines.
Figure 1 shows different simulated trips between two stations. Each point is a trip, and is characterised by its running time and its energy consumption. The flat out trip has the minimum running time and the maximum energy consumption. It can be seen that different trips with different energy consumptions can provide the same running time. Thus the optimum solution from the energy point of view is the trip located on the Pareto curve.
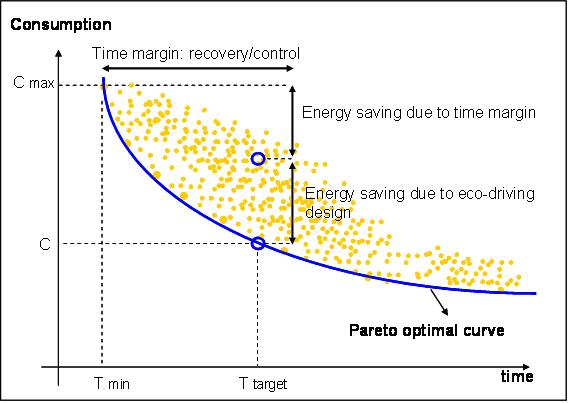
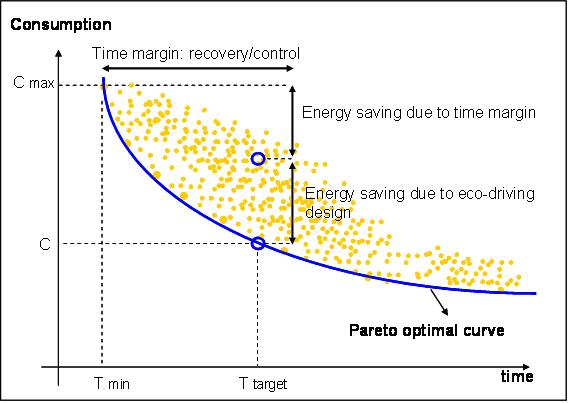
Figure 1 The different simulated trips between two stations
Once the running time has been chosen in the design of the timetable, energy savings can be obtained using the time margin of the timetable. Subsequently, the driving must be designed to obtain the minimum consumption associated to that running time (eco-driving design). Eco-driving consists of a sequence of efficient driving commands: coast, speed regulation and speed regulation without braking. Finally, the slot assigned to the service designed must be respected. That is, the driving of a train should not affect the following train on the same line.
Once the timetable and the eco-driving have been designed, traffic control allows realtime planning and driving updates when the train is delayed. Thus, to adapt the driving to real-time changing conditions, a new ecodriving is put into practice with a different travel time.
Three levels can be distinguished in rail traffic control:
1. Single train regulation. The schedule is pre-programmed and onboard train control is necessary if the schedule is to be complied with.
2. Re-scheduling. The schedule is modified to adapt the driving in real-time to non-planned speed reductions or important delays.
3. Global traffic control. The centralised regulation system allows global traffic optimisation, and the minimising of delays and of energy consumption (on-line communication).
The optimal solution is automatic traffic regulation and execution of commands, supervised by the onboard signaling system. In metro lines with a global traffic control and equipped with ATO systems that execute efficient commands, important energy savings and improvement in regularity can be achieved (measurements in Metro de Madrid: 18% of average energy savings and an improvement of 50% in regularity).
On high-speed trains, it is necessary that the eco-driving can be modified on line according to the changing conditions, such as temporary speed limits or delays, by means of onboard advice systems or efficient ATO systems.
The railways systems research group at the Universidad Pontificia Comillas has developed a combined model for timetable and eco-driving design and applied this model to study cases on Spanish high-speed lines. The eco-drivings designed have been tested on commercial services and energy savings have been measured. The model is based on accurate simulation of the eco-driving design for each track section between consecutive stops. Different trips on the same section of track are simulated by executing different efficient driving command sequences, which result in different running times and associated consumption. The optimal Pareto curve is obtained as the minimum consumption point for each running time. Once the Pareto curve has been calculated for all the track sections, these are inputted to the timetable design model, a mathematical optimisation model that calculates the optimal distribution of time margins and the corresponding optimal timetable.
Renfe and Comillas have been collaborating in different projects to design eco-driving for the following cases: high-speed train (HST) class 102 in high-speed line (HSL) Madrid–Barcelona (Madrid–Zaragoza section), HST class 120 in HSL Madrid–Barcelona (Madrid–Plasencia de Jalón section), HST class 103 in HSL Madrid–Barcelona, HST class 102 in HSL Madrid–Málaga, and HST class100 in HSL Madrid–Sevilla.
Given that the objective is to obtain realistic designs for efficient driving that can be later be put into practice on commercial services, it is essential that an accurate and reliable simulator be developed. Thus a detailed model of the infrastructure, the train and the driving must be taken into account in the simulations (see Figure 4).
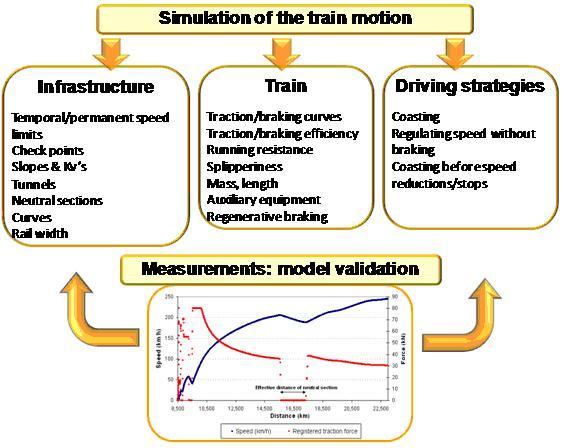
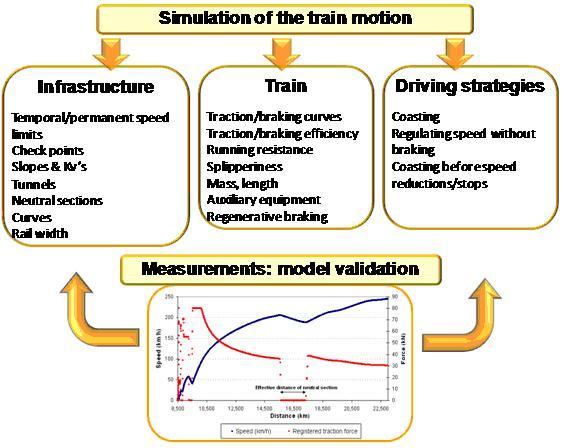
Figure 4: A detailed model of the infrastructure, the train and the driving must be taken into account in the simulations
Once the simulation model had been developed, preliminary night-time tests and tests on commercial services were carried out for model adjustment and validation. Measurements were recorded onboard by means of recording equipment connected to the traction system communication bus. The onboard measurements were recorded every 250ms, including traction/braking force, consumed/regenerated current, voltage, speed and distance. Nocturnal specific tests were carried out. These included coasting from 300km/h to stop to calculate running resistance, regulating different values of constant speed, and driving at constant traction force to measure the efficiency of traction equipment. ADIF (the Spanish Railways Infrastructure Administration) collaborated in these nocturnal tests, recording consumption measurements at substations.
Commercial services were also analysed to obtain information about non-guided driving (before the design of eco-driving), and manual driving models. From these validation tests, the following elements of the model were adjusted: the traction/braking curves; the traction/braking efficiency; the consumption of auxiliary equipment; the length of neutral sections of the traction network; the manual driving patterns; and the electrical losses (these, measured during nocturnal tests comparing onboard train and substations measurements, were about 4%).
Once the simulator had been validated, the eco-driving was designed for the selected line, train and commercial service, and finally, the designed eco-drivings were tested. Eco-driving was guided according to the optimal design in commercial services and onboard measurements were recorded to compare them with non-guided driving measured in the same trip conditions (non-delayed trains).
Eco-drivings have been tested in HSL Madrid–Barcelona with HST classes 102 and 120 (Talgo and CAF high-speed trains). For this purpose, 19 tests with HST in commercial services and 12 specific tests were carried out from July to December 2009.
Energy savings were up to 70% on the Guadalajara–Madrid section, compared to other non-guided trips which departed punctually on the same section, and up to 42% on the Calatayud–Zaragoza section. HSL Madrid–Barcelona was used in both sets of tests. Average savings measured on this line were 21%.
This shows the impact that strategies focused on traffic operation can have on the reduction of energy consumption, particularly if the possibility of its application in the shortterm and with a low effort and investment cost is taken into account.
In addition, the simulator validated in the tests conducted can be used to explore new possibilities in energy efficiency, making use of technologies to use regenerated energy, onboard energy storage devices / feed auxiliary systems, energy transmission between trains, fixed energy storage devices, and reversible substations. Thus valuable information for the analysis of energy utilisation in relation to the associated investment can be obtained. The efficiency of each technology can be analysed individually and in combination with others, taking into account train frequency and electric net characteristics.
The proposed procedure is based on the calculation of efficiency factors for every scenario to be analysed. For each simulated traffic scenario (for example, the existence of an accumulator in the substation in addition to an onboard accumulator), energy consumption and regenerated energy is calculated at the substation to take into account electrical losses and compared to the energy regenerated by the train. The power flow model is solved first without considering the regenerated energy. Subsequently it is solved once more, but this time taking into account the selected scenario (the specific technology and traffic, that is, the headway between trains). Thus, average energy savings can be calculated for the corresponding combination of technologies.
The efficiency factor, which measures the average use of the regenerated energy, can be calculated as shown in Figure A, where ΔEsubstation is energy saving in the simulated scenario measured on substation energy meters, and Ereg,pantograph is total regenerated energy measured on train pantographs.
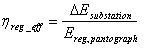
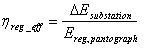
Figure A
Then, the energy consumption at substation for a single train can be estimated as shown in Figure B, where Econsumed,pantograph is the energy consumed by trains measured on the pantographs, and ηlosses is the ratio of electrical losses in catenary.


Figure B
Depending on the traffic conditions (headway between trains) and the combination of technologies, the improvement in the use of the regenerated energy will vary, so the associated energy saving may or may not justify the investment in these technologies. Of course, if this efficiency factor is used, the ecodriving design should be modified and the resulting optimal driving will consist of a new sequence of commands with an associated change in consumption. That is, the simulated trips and the resulting Pareto curve show a reduction in consumption, and the selected driving for a specific running time will not be the same as the optimal driving without considering the factor.
Finally, it can be concluded that energy efficiency on railways is not only a matter of the long-term strategic planning of new lines and the designing of new trains, but also of shortterm, low-investment traffic operation measures designed to reduce consumption. These strategies, which optimise driving and the design of timetables, can have an important impact on the efficiency of rail systems and have been tested on Spanish highspeed lines and Renfe trains, obtaining an average energy saving of 22%.
About the Authors
Paloma Cucala García
Paloma Cucala García is a tenured Assistant Professor of the Comillas’ Engineering School, ICAI and a Research Fellow in the Institute for Research in Technology. She is the Coordinator of the Rail Systems Research Group of the Institute and the Coordinator of Comillas’ Master’s Degree in Rail Systems. She has been working on research projects in collaboration with the railways industry and administrations, especially on energy efficiency and optimisation of capacity and traffic control.
Antonio Fernández Cardador
Antonio Fernández Cardador is a tenured Associate Professor of the Comillas’ Engineering School, ICAI and a Research Fellow in the Institute for Research in Technology. He is a member of the Rail Systems Research Group of the Institute and the Director of Comillas’ Master’s Degree in Rail Systems. He has been working on research projects in collaboration with railways industry and administrations, especially on energy efficiency and optimisation of capacity and traffic control.